Magnetic refrigeration
Almost a century of demagnetization cooling
Magnetic refrigeration is a well-established technique that was proposed and first implemented back in the 1930s. It can be used to generate sub-Kelvin temperatures by exploiting the magnetic field dependence of the entropy of a spin system. This is illustrated in the following graph, which shows the entropy as a function of temperature for an ideal spin ½ system.
At high temperatures, the spins are randomly oriented and their entropy is constant. In contrast, at low temperatures, the entropy of the spin system is a function of the magnetic field B and the temperature T. In this regime, reducing the magnetic field from point (B) to point (C) under adiabatic conditions – i.e., while keeping the entropy constant– will result in a decrease of the temperature. For this reason, magnetic cooling is also known as “adiabatic demagnetization refrigeration,” or ADR for short.
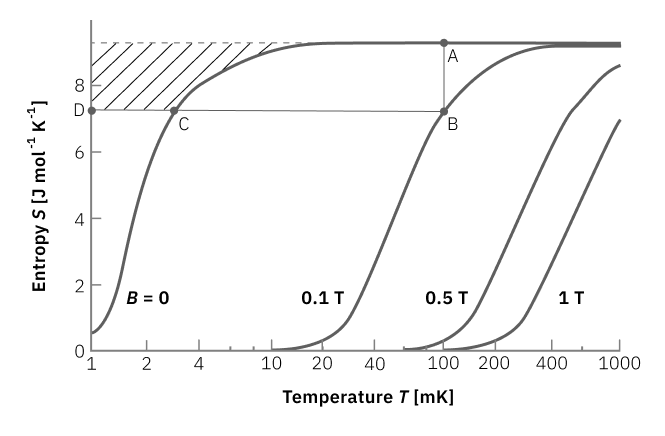
ADR – Adiabatic Demagnetization Refrigeration
How magnetic cooling works
A typical ADR configuration consists of a pre-cooling unit, typically a closed-cycle cryocooler, a heat switch, a superconducting magnet surrounding a solid-state cooling medium, and a sample platform.
At the beginning of the ADR process, the heat switch connecting the ADR with the pre-cooling unit is closed and both the cooling medium and the attached sample stage are cooled to the cryocooler base temperature of about 4 K.
While the heat switch is still closed, the cooling medium is magnetized by driving a current into the superconducting coil. The heat of magnetization is released and dissipated in the main thermal bath provided by the cryocooler.
Therefore, after the maximum magnetic field has been reached, the cooling medium will return to its base temperature. The entropy of the system is now essentially determined by the magnetic contribution of the spin sub-system of the cooling medium.
Then the heat switch is opened to break the thermal connection between the pre-cooling unit and the cooling medium. By reducing the magnetic field adiabatically, the temperature of the cooling medium drops thereby cooling the attached sample stage.
Usually, the medium is not completely demagnetized. Instead, the magnetic field is tuned to settle a target temperature and the remaining magnetic field is used to compensate for heat leaks from radiation, the support structure and wiring, as well as heat generated by the experimental setup. Thus, by further reducing the magnetic field the sample temperature can be stabilized with high precision for a limited period of time, the “hold time,” which is typically several hours.
Once the magnetic field has been reduced to zero, the ADR process can be repeated. The time to regenerate, i.e., to re-magnetize and relax the cooling medium will usually be 1-2 hours, resulting in typical duty cycles of 70 to 95%.
cADR – continuous Adiabatic Demagnetization Refrigeration
How to provide long-lasting sub-Kelvin temperatures without cryogens
To provide continuous cooling without cryogens, we consider a system with two ADR units, where, similar to the conventional “one-shot” ADR configuration, the first unit is connected to the main thermal bath via a heat switch. But this time a second ADR unit is added. It is connected on one end to the first ADR unit using another heat switch and on the other end to the sample stage.
As shown schematically, the two interconnected ADR units will work together to extract heat from the sample stage and to pump it into the 4 K main thermal bath.
Besides this serial two-stage configuration, many different cADR layouts with multiple ADR units can be realized, including serial or parallel configurations and even combinations thereof.
The optimal configuration depends on the demands of the experimentalist and the specific experimental conditions – our tech team is happy to support you in choosing the best solution for your purpose.
Both the first and the second ADR unit are fully magnetized and connected through their respective heat switches. Therefore, the cooling media and sample stage initially have the temperature provided by the cryocooler.
Unit 2 is decoupled from unit 1 and demagnetized, thereby cooling the sample stage down to the target temperature.
Unit 1 is disconnected from the bath and demagnetized, too. It stabilizes a temperature well below the setpoint temperature of unit 2. Now the heat switch between the two ADR units is closed and unit 2 can be regenerated, while at the same time the temperature of the sample stage is kept constant.
After unit 1 is depleted, the connection between the two units is opened again by opening the corresponding heat switch and unit 1 is regenerated at the temperature of the cryocooler.
This procedure can be repeated infinitely to provide continuous cooling at the sample stage.
Automatic operation
Turnkey refrigerators
At kiutra, we believe that innovation and user-friendly design should be an essential part of cryogenics. We are devoted to manufacturing highly automated cryostat systems that can be operated without expert knowledge.
Because they don’t require liquid cooling media in the cooling process, our cryostats are very robust and fully automatic.
All systems can be controlled using a modern, user-friendly graphical software based on an open-source Python framework that can easily be adapted to your preferred measurement equipment and setups.
Automatic Sample Changer
An outstanding feature developed by our cryo-engineers is the Automatic Sample Changer, a top-loading load-lock mechanism supplied as part of our L-Type Rapid cryostat. The mechanism enables you to install sample pucks and cool them down to base temperature in less than three hours.
The Automatic Sample Changer offers several benefits: When using several sample pucks a number of users can prepare their experiments in parallel. This is helpful if the preparation is complex and would block the operation of conventional systems and cause significant downtimes.
The sample loading process is extremely fast.
It takes just seconds to plug a sample puck into the transfer cage and secure the latter inside the vacuum lock and a couple of minutes to automatically load the puck to the low temperature stage, where it is cooled to the base temperature in less than three hours. The fast-cooling pucks are particularly useful for the high-throughput screening of material samples and to accelerate the development of cryogenic devices.
As the sample preparation and sample loading does not involve any manual work at the cryostat or fragile probe sticks. Using the sample changer and sample pucks is an ideal solution for less experienced users, shared facilities, and in industrial environments.
Glossary
Heat switch
A heat switch is a tunable thermal link. Heat switches are widely used in cryogenics and are a key component for every magnetic refrigerator.
In a magnetic refrigerator, a heat switch is used to decouple the ADR cooling medium from its pre-cooling stage during the demagnetization cooling. The heat switch is closed to conduct the heat of magnetization, which is generated during the regeneration process, into the pre-cooling stage.
There are several types of heat switches, each with different advantages and disadvantages. The most common types are mechanical switches, gas-activated heat switches, and superconducting heat switches. Another distinction is between active and passive heat switches. With active heat switches, the switching process can be controlled by the operator. With passive heat switches, the switching process always happens at a certain, pre-defined temperature.
Hold time
With a “one-shot” ADR cryostat, the cooling medium can provide cooling only for a limited amount of time, the “hold time”, which is determined by the cooling capacity of the medium.
After the cooling medium is magnetized and the heat of magnetization has been dissipated in the pre-cooling stage, the heat switch is opened and the cooling medium is partially demagnetized. During demagnetization of the cooling medium its temperate drops. Once the target temperature has been reached, demagnetization of the cooling medium continues at a smaller field ramp rate, which is set to compensate for thermal leaks through the support structure, thermal radiation, or customer setups. The total time available at the target temperature is referred to as “hold-time”. It ends once the magnetic field has been reduced to zero, i.e., when cooling medium is fully demagnetized and the cooling process terminates.
The hold time will vary as a function of temperature, so typically, when the system is operated at the lowest temperature, the system may still be operated at higher temperatures by re-magnetizing the cooling medium.
A major benefit of magnetic temperature control is that temperatures can be stabilized with high precision, as no valves, capillaries, or heaters are involved.
One-shot
“One-shot” operation refers to the fact that conventional ADR cryostats can provide cooling only for a limited amount of time, the “hold-time”, after which the cooling medium must be regenerated. The regeneration process takes, depending on the cooling medium, 1-2 hours.
When regenerating, the cooling medium is typically warmed up to slightly above the temperature of the pre-cooling stage. As the “hold-times” of “one-shot” ADR cryostats are several hours, duty cycles between 70 and 95% can be achieved.
Our multi-stage, cADR cryostats for the first time provide a commercially available continuous magnetic cooling solution.
Stray fields
A common concern regarding magnetic refrigerators is the presence of magnetic stray fields at the sample position when the cryostat is running. At kiutra, we pay special attention to these magnetic fields, to enable measurements of very sensitive materials and devices.
In all our cryostats, we use compensated superconducting magnets to reduce the stray fields at the sample position and to minimize the magnetic interference between neighboring cooling units in multi-stage systems.
In our L-Type Rapid, we maximized the distance of the ADR magnets and the sample stage to minimize the stray field .Together with our compensated magnet coils this ensures stray fields of less than 50 µT at the sample position, which is similar to the earth’s magnetic field. As in any other cryostat, mu metal shielding can be used to further reduce these small residual fields. This makes the L-Type Rapid a suitable tool to probe sensitive materials and devices, such as superconductors or quantum electronics.
It is also noteworthy that the stray fields given in our specifications always refer to the maximum magnetic field in the cooling unit. In one-shot ADR cryostats, however, measurements are usually performed with the magnet operating at 0-30% of its maximum field, resulting in accordingly reduced stray fields. Similarly, in cADR systems, most of the magnets are operating at 10-30% of their maximum charge, which again leads to a reduction of the effective residual fields at the sample position.
Our R&D projects
We are an active contributor to a growing number of cryogenics and quantum technology research projects
MILLENION QUANTUM
kiutra is providing the cooling platform for the MILLENION project, a collaboration of 14 academic and industrial partners across Europe to advance trapped-ion quantum computers.
MUNIQC-SC
We are part of the MUNIQC-SC consortium, an initiative to build a quantum computer demonstrator based on superconducting circuits.
SPROUT
Together with Delft Circuits, kiutra has received funding from the European Eurostars program to develop a scalable platform for Quantum Technologies.
MATQu
We are part of the Materials for Quantum Computing (MATQu) consortium, which was set up to establish a European value chain for superconducting qubits.
EIC Accelerator Pilot
Supported by the prestigious EIC Accelerator programme kiutra is developing a unique characterization tool for qubits and related low-temperature electronics.
MARQUAND
The MARQUAND project was a joint effort of the Walter-Schottky Institute and kiutra. Its goal was to develop and operate highly efficient quantum detectors.